EDITING WITH CRISPR
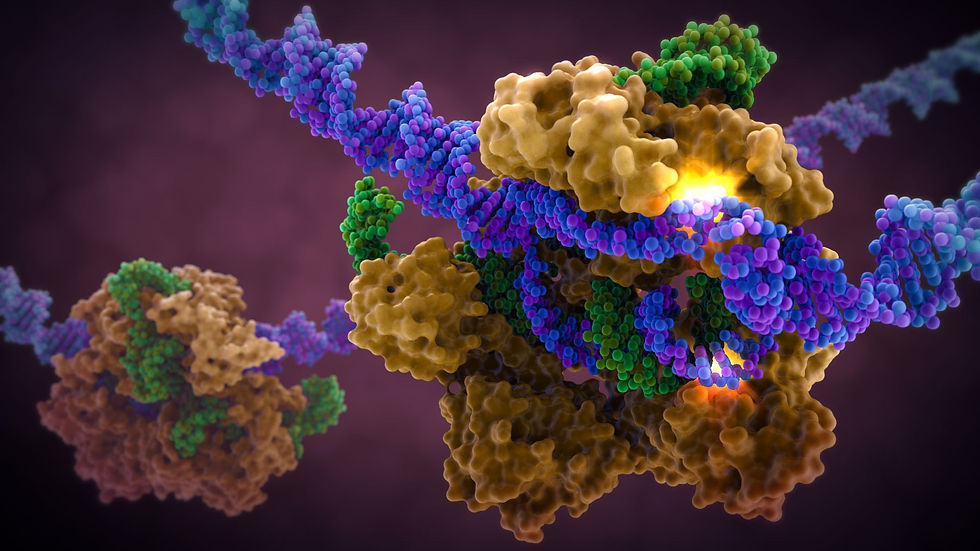
[ECI1]
GENE KNOCKOUTS
CRISPR is widely known and used to knock out specific genes in an organism's genome for their own benefit. The most common mechanism used for knockouts is the non-homologous end joining (NHEJ) repair pathway. In this procedure, the guide RNA leads the Cas nuclease to the targeted DNA locus and performs a double-strand break (DSB). This break is then repaired by NHEJ, which can either lead to the deletion or insertion (indel) of nucleotides in a DNA sequence. Consequently, frameshift mutations can occur due to the alteration of the sequence, especially if it is not done perfectly.

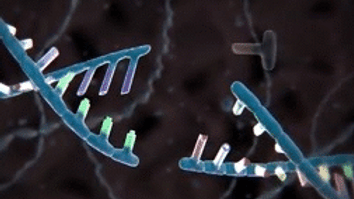
Cas nuclease
DSB
NHEJ Repair
frameshift mutation
guide RNA
[ECI2]
[ECI3]
[EC1]
GENE KNOCK-INS
Unlike knocking out specific nucleotides in a DNA sequence, the knock-in experiment edits the targeted genome by inserting a new, usually altered, DNA fragment called template DNA. With this experiment, however, scientists need to redirect the cell from using its usual NHEJ repair pathway to a more specific and sophisticated mechanism called homology-directed repair (HDR) pathway. In this process, the cell duplicates the genetic sequence of the new DNA in order to precisely replace the segment that was broken because of the DSB.
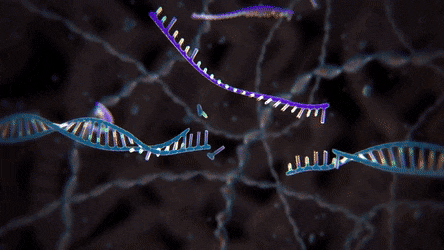
duplicated genetic sequence
[ECI4]
[EC1]

[ECI9]
IMPORTANCE OF PAM SEQUENCE
The photospacer adjacent motif (PAM) is a 2-6 base pair DNA sequence that follows the DNA sequence targeted for cutting by the CRISPR-Cas9. What makes PAM so crucial in all of CRISPR experiments is that PAM is the sequence that ensures Cas9 does not get triggered to cut off some of its own bacterial genome. Whenever the Cas nuclease cuts a viral DNA, it stores its remains in the CRISPR spacers, but excludes the PAM sequence in order for the bacteria to not recognize its own genome as a target.
​
When scientists design and modify their own gRNA for CRISPR experiments, whether it is knockingout or knocking-in genes, they take out the PAM from the guide RNA. This is because the gRNA will be automatically cut by the Cas nuclease if it contains the PAM sequence.
[EC2]
DIFFERENT Cas NUCLEASES
1. Cas9
from the bacterium Streptococcus pyogenes (spCas9)
Has two parts of the protein: the recognition part and the nuclease part. The former finds and senses the targeted DNA, while the latter has nuclease domains which cut the DNA (DBS). However, it has a few limitations: the Cas9 holds the risk of cutting DNA sequences that are not targeted, its large size makes it challenging to transfer the nuclease inside cells, and the need for the PAM sequence limits the manipulation of the target DNA.
2. Cas12a
(Cpf1)
CRISPR from Prevotella and Francisella 1 hence Cpf1
Unlike the Cas9 nuclease, Cpf1 makes a double-strand cut that makes the end sticky, which is convenient for experiments that only focus on HDR and adding genes. Additionally, it is smaller than Cas9, only requires a short strand of guide RNA, and recognizes slightly different PAM sequences. However, when it cuts the targeted DNA (DBS), it also automatically cuts non-targeted single-stranded (ss) DNA.
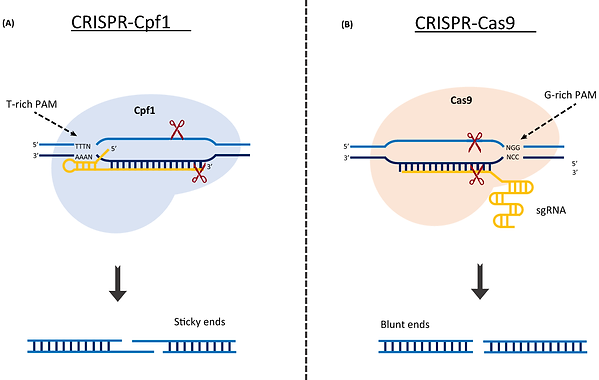
2. Cas14a
from archaea bacteria
Cas14a does not require PAM sequence, targets single-stranded (ss) DNA, and cuts other non-targeted ssDNA when binding the target sequence, like Cas12a. Unlike Cas12a, Cas14a is quick to detect mismatches when cutting the targeted ssDNA, which makes it a perfect method in diagnostics.
3. eSpCas9
eSpCas9 is an enhanced nuclease that is more accurate than the widely known Cas9. Based on its structure, researchers hypothesized that instead of cutting the double-stranded DNA, the strands just separate as the RNA binds to the targeted DNA strand. The nuclease has a deep fall, lined with positive charges that are thought to neutralize the DNA strand that is negatively charged. In addition, it is proven to be more accurate and also have a low off-target editing rates.

4. xCas9
xCas9 is a genetically engineered nuclease that can now recognize a wide range of PAM sequences, which increased the target sites from 1 in 16 to 1 in 14 in the genome. With this new nuclease, it solved the problem of the limited enzyme activity. xCas9 also demonstrated to have lower off-target rates compared to SpCas9.
5. Cas13a
from the bacteria Leptotrichia shahii
Instead of targeting the DNA which causes a permanent damage to the organism's genome, Cas13a targets the RNA instead. It contains 2 ribonuclease that speed up the maturation of CRISPR RNA and also the degradation of single-stranded RNA in bacteria.
[ECI5]
[ECI6]
[EC3]
STEPS ON HOW TO USE CRISPR
[EC4]
01
DESIGN
Design gRNA for CRISPR in order to find the targeted DNA sequence. Edit the guide RNA based on whether it is going to be used to delete (knockout) or add (knock in) genes into the sequence.
02
EDIT
When gRNA is ready, it can precisely edit the targeted DNA sequence after the proper CRISPR enzyme is selected, while taking into consideration the PAM sequence that is found in the target DNA sequence. Deliver components with the best transfection method.
03
ANALYZE
Analyze and observe the results of the sgRNA on the DNA sequence, especially after being edited. Plan for the next experiment by reviewing the procedures and the outcomes.
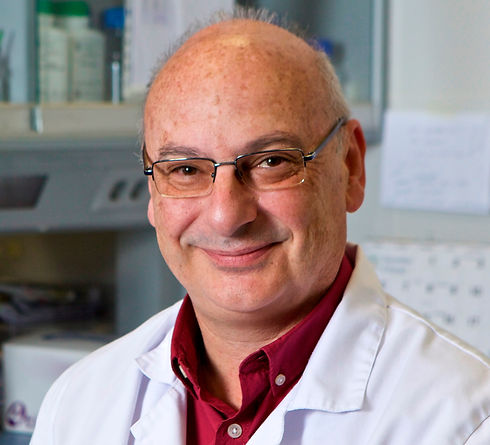
What is your favorite CRISPR application?
Well, everyone is worried about health, but as a microbiologist I am biased. For me, one of the most interesting applications is as antimicrobials to kill bacteria. These tools can be programmed to specifically target the DNA of bacteria that are pathogens. With all its advantages, for example, in the gut microbiota – if you take an antibiotic you kill all the other beneficial bacteria in the gut.
[EC5]
- FRANCISCO MOJICA
microbiologist known for his research on CRISPR
[ECI7]
